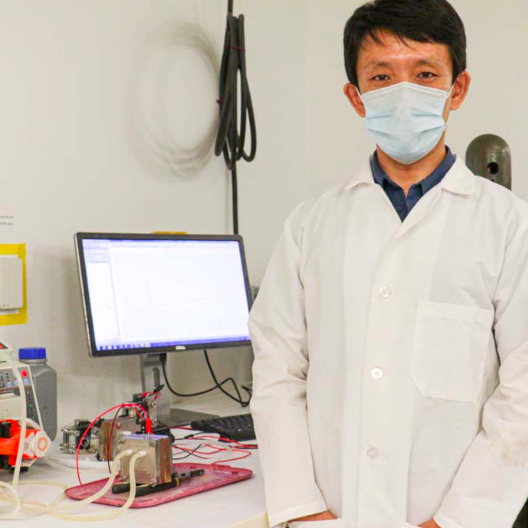
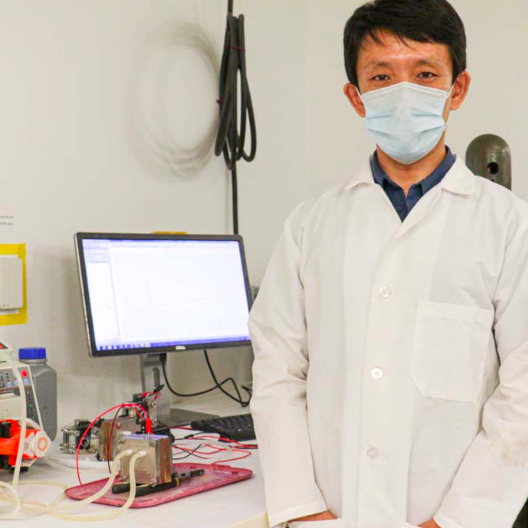
Research Terms
Researchers at the University of Central Florida have invented a heterostructured catalyst that increases the energy harvesting capabilities of solar cells. Besides enhancing solar cells and solar fuel cells, it can also function as a photocatalyst for water splitting—the chemical reaction that breaks down water into oxygen (O) and hydrogen (H2). It thus enables a cheaper, more efficient way to produce hydrogen fuel from seawater.
The heterostructured catalyst exhibits enhanced light absorption in a broad range of the solar spectrum, from the ultraviolet (UV) light region to the visible and near-infrared (IR) light regions (generally in a range of 300 nm to 700 nm). Developed using low-cost, nontoxic, and chemically stable titanium dioxide (titania, TiO2), the technology works without the need for heavy doping, narrow band gap semiconductors, or costly and potentially environmentally toxic noble metals and co-catalysts.
Technical Details
The UCF technology comprises a thin film heterostructured catalyst, methods for forming the catalyst, and using the catalyst. One method includes anodizing a titanium surface on a substrate to form a 2-dimensional (2D), periodically ordered array of titania (TiO2) nanocavities. A metal layer or a metal compound layer on the titania nanocavities follows. The substrate can be a silicon wafer, a glass wafer, or a conducting polymer. Another method converts the titania nanocavities into strontium titanate (SrTiO3) nanocavities. The metal layer or metal compound layer on the inner surface of each nanocavity wall can be molybdenum disulfide (MoS2), SrTiO3, or a variety of different metals or metal alloys. Nanopore size can be 10 to 200 nm with a nanowall thickness of 5 nm to 20 nm.
Partnering Opportunity
The research team is looking for partners to develop the technology further for commercialization.
Stage of Development
Prototype available.
MoS2/TiO2 Heterostructures as Nonmetal Plasmonic Photocatalysts for Highly Efficient Hydrogen Evolution, Energy & Environmental Science, 2018,11, 106-114
Researchers at the University of Central Florida have developed an inexpensive battery cell that overcomes the safety hazards, performance problems and capacity loss associated with using lithium metal as an anode electrode in lithium-ion (rechargeable) batteries. The UCF invention includes a novel semi-liquid coating that forms a stable, evenly distributed solid electrolyte interface (SEI) buffer between the electrolyte layer and the surface of the lithium metal anode.With the innovation, manufacturers now have the technology to produce next-generation energy storage devices as well as better lithium-ion batteries.
The invention comprises a battery cell with an inexpensive buffer layer (coating) and a method of applying it onto a lithium metal electrode surface. Made of a semi-liquid gallium-indium-tin eutectic alloy, the buffer forms a stable and conformal interfacial layer that deters dendritic growth and protects the electrolyte during charging/discharging cycles. Since the alloy is in a liquid state at room temperature, it can maintain good contact with the lithium metal surface. Also, by solidifying in the presence of lithium ions and liquefying in the absence of lithium ions, the SEI allows the battery to safely achieve coulombic efficiency over multiple cycles without losing capacity.
The research team is looking for partners to develop the technology for commercialization.
Prototype available.
University of Central Florida researchers have developed a method to produce efficient, cost-effective, and bifunctional (that is, anodic and cathodic) catalyst for water electrolysis applications. The new catalyst is a quarternary iron/nickel phosphoselenide nanoporous film (FeNi-PSe NF) with superior electrocatalytic properties that enable hydrogen evolution reaction (HER) and oxygen evolution reaction (OER).
Example applications include hydrogen and oxygen generation from alkaline solutions, deionized water, and seawater. Currently, hydrogen production results from steam methane reforming and coal gasification, demonstrating a heavy dependence on fossil fuels. The goal of shifting to large-scale hydrogen production is constrained by costly platinum group metal (PGM) catalysts.
With the invention, the researchers synthesized the NFs using electrochemical bottom-up (electrodeposition) and top-down (anodization) processes followed by a chemical vapor deposition (CVD) method. In the inventive group’s studies, the catalysts show an electrolysis efficiency of 69.7 percent, approximately 10 percent higher than other reported state-of-the-art catalysts.
Technical Details
The UCF invention is a method of synthesizing a bifunctional catalyst for water splitting applications. By modifying the electronic structure and composition of the catalyst, the method produces a self-supported quaternary iron/nickel phosphoselenide nanoporous film (FeNi-PSe NF). The slightly oxidized FeNi-Pse NF surface serves as an active site for oxygen evolution reactions, making hydrogen evolution reactions and oxygen evolution reactions well-balanced, thereby improving electrolysis efficiency.
The process of forming an iron-nickel-phosphorus nanofilm can comprise the following:
The selenium stabilizes the catalyst and improves its electrical conductivity. Multiple pores formed through the quaternary iron-nickel phosphoselenide nanoporous film improve the transportation of mass through the film.
In one example application, the FeNi-PSe NF works as an anode and a cathode in a two-electrode electrolytic cell. When the cell is subjected to a water source, such as seawater, the NF splits the water molecules in the water source. The hydrogen evolution reactions convert the water into hydrogen fuel that is usable as a renewable energy source.
Partnering Opportunity
The research team is looking for partners to develop the technology further for commercialization.
Stage of Development
Prototype available.
The University of Central Florida invention is a universal strategy for designing a high-performance, safe, alloy anode for aqueous (water) batteries. Metal anode instability, including dendrite growth, metal corrosion, and hetero-ions interference, occurring at the electrolyte/electrode interface of aqueous batteries, are among the most critical issues hindering their widespread use in energy storage. Zn-M alloys are used as model systems (M = Mn + other transition metals). Experiments have achieved stability over thousands of cycles even under harsh electrochemical conditions, including testing in seawater-based aqueous electrolytes and using a high current density of 80 mA cm^-2.
The University of Central Florida invention is a design strategy for discovering new materials and catalysts for energy conversion. The method seeks to regulate the local bonding and local coordination environment (LCE) of the classic metal-nitrogen-carbon (M-N-C) catalysts by infusing fluorine (F)-coordination. As a result, the rationally designed catalyst shows a maximum power density of 0.57 W cm-2 and more than 5,900 hours of operation in direct ethanol fuel cells, outperforming benchmarking catalysts. The tactic of F-induced LCE regulation can be applied to other catalysts with drastically improved activities and stabilities.
The University of Central Florida invention provides freestanding, nanoporous thin films (NPFs) to help meet the challenges in renewable energy production and conversion applications. With its unique electrodeposition process, the UCF technology uses low-cost, earth-abundant resources such as iron (Fe), cobalt (Co) and nickel (Ni).
Traditional energy conversion and storage materials comprise powders and particles that require organic binders and current collectors for electrode assembly. However, the organic binders in the materials increase electrode resistance and complicate material recycling. The physical deposition methods for growing thin films (such as sputtering, evaporation, and epitaxy techniques) make it difficult to control the nanostructure. Also, the substrate on which the thin film forms is not easily removable, thus limiting the film’s applications.
As a solution, the UCF invention does not require organic binders or current collectors. The invention offers better control over the nanostructure growth and enables easy removal of the NPF from the substrate, which can then be recycled. Applications for the material may include electrodes in catalysis, supercapacitors, lithium batteries, fuel cells, hydrogen storage, CO2 storage, separation and sensing, seawater desalination, writing heads, compact disks, and shielding foil.
Technical Details
The UCF invention consists of freestanding NPFs and a process for producing the NPFs and layers. The process results in unique nanoporous structures through the electrodeposition of Fe, Co, Ni, and their alloying combinations (such as NiFe, NiCo, FeCo, NiFeCo). The thin films can be further enhanced by treating the deposit layers with anodization or, in some cases, chemical vapor deposition. One of the advantages of the chemical deposition processes is that certain metals (such as iron) may be deposited onto the substrate without oxidation.
In one embodiment, the electrodeposition process involves submerging a substrate in an electrochemical deposit bath with a metal salt and saccharin. The time that the substrate remains in the bath depends on the desired thickness of the NPF. In the example, the nanoporous and flexible film has pores with about 20 nm diameters formed after anodization. In an alternative embodiment, the pore sizes may range from 15-25 nm. Alternatively, pore sizes may range from 18-22 nm.
Partnering Opportunity
The research team is seeking partners for licensing, research collaboration, or both.
Stage of Development
Prototype available.