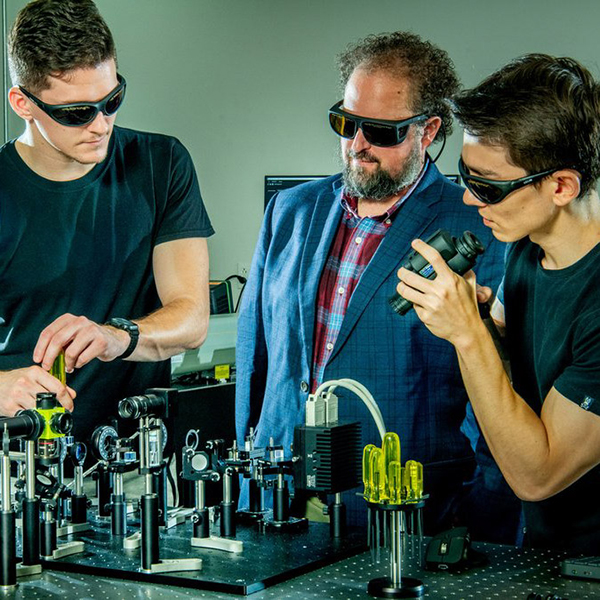
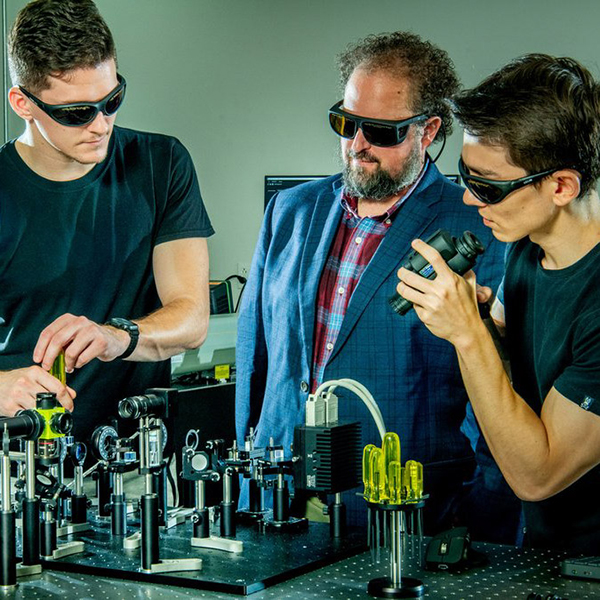
Research Terms
This radiographic monitoring system tracks particle beam delivery within a patient undergoing particle therapy. The goal of radiation therapy is to maximize the treatment dosage delivered to a tumor while minimizing exposure of surrounding healthy tissue. Studies have shown that charged particle therapies such as proton therapy and heavy ion therapy significantly reduce toxicity to normal tissue while focusing radiation on a tumor. However, real-time guidance in charged particle therapy is limited by the finite range of the charged particles, which makes it difficult to determine the precise position of the tumor target within the beam aperture.
Higher energy particles during the interaction with matter can produce secondary particles such as neutrons, electrons, x-rays and gammas. Neutrons and photons, which tend to be more penetrating than the charged particles, typically give the largest contribution to the secondary dose in out-of-field organs. Researchers at the University of Florida have developed a portal imaging system for charged particle therapy based on real-time image acquisition and in situ dose monitoring. It uses the exit neutrons and photons generated within the patient during treatment to pinpoint where the beam source targets and measure the dosage applied to the region.
Portal imaging system to produce radiographic images that improve particle therapy dosing at targeted regions
First-of-its-kind proton portal imaging system that provides “beam’s eye view” or portal of patient anatomy. This particle therapy portal imaging system provides radiographic images of a patient anatomy. When a charged particle beam consisting of high-energy ions lands on a target, it generates a secondary exit neutron and photon flux. The imaging system uses these neutron and photon emissions within the irradiated body to generate radiographic images of the patient. These images inform accurate assessment of dosage delivery to a tumor target and surrounding anatomical structures.
These RANA batteries carry out more efficient energy conversion than available betavoltaic batteries and their long lifespans allow them to power implantable medical devices, including pacemakers. Like other nuclear batteries, they include a radiation source that works with other materials to convert decay energy into an electric current. While the typical betavoltaic battery has a layered structure of alternating plates of radioisotopes, decay energy converters, and photovoltaic (PV) cells, these new batteries, developed by University of Florida researchers, do not require alternating sheets. The RANA batteries are conformable, easy to manufacture, and offer more efficient energy conversion, making them attractive components for biotechnology applications. The lifespan of an in vivo bioimplant fitted with a RANA battery can exceed one hundred years.
Nuclear batteries that carry out more efficient energy conversion to power implantable medical devices, including pacemakers
Researchers at the University of Florida have developed more efficient nuclear batteries by encapsulating select radioactive nuclides within materials that can either convert or multiply radioisotope decay emissions into photons, which then interact with organic photovoltaic (OPV) cells. The OPV electricity generation mechanism is similar to conventional solar cells with the exception that the photons are initiated by the decay of a radioisotope. Depending on the source radionuclide, the decay process used in these batteries can be beta (electron emission) or gamma (photon emission). Doping the radioactive beta or gamma emitters directly into the photon conversion material and dispersing the particles into a translucent medium eliminates several of the major efficiency loss mechanisms found in traditional multi-layered designs.
Related to 14778
This concrete formulation and or cementitious coating contains boron to protect against radiation-induced volumetric expansion. Many facilities use concrete to shield against radiation, such as nuclear power plants or radiological medical facilities. The global nuclear power plant and equipment market is projected to reach $4.9 billion by 2025. Concrete is prone to degradation in certain environments, a result of chemical reactions between alkalis and other materials in the concrete mixture. Some concrete formulations use boron aggregates to make concrete structures resistant to radiation, but the inherently uneven aggregate dispersion leaves surfaces exposed and the structure susceptible to radiation-induced volumetric expansion (RIVE), which may lead to further degradation due to alkali silica reactivity (ASR) of aggregates that are otherwise non-reactive.
Researchers at the University of Florida have developed a formulation for concrete or concrete coatings to help prevent degradation due to radiation. The formulation utilizes boron compounds that decay into lithium, which can suppress the expansive chemical reactions that cause degradation.
Boron-containing compounds in concrete and cementitious coatings to protect against radiation damage and increase concrete’s durability and service-life
Concrete formulations often use lithium salts to reduce the potential for alkali silica reactivity degradation and boron aggregates to provide neutron shielding. This formulation includes boron-containing compounds, such as fine powders, liquid solutions, or liquid suspensions, in the mixture of cementitious materials. When exposed to neutron radiation, the boron transforms in situ through radioactive decay into lithium. The formulation also contains hydrogenous compounds to aid in providing shielding from thermal neutrons as well as the thermalization of fast neutrons for additional shielding benefits. This protects the radiation-damaged aggregates and prevents alkali-silica reactions due to radiation-induced volumetric expansion. A shielding coating for concrete using this formulation can be applied directly to a surface prepared for bonding or mechanically as pre-formed panels that secure onto an existing structure. The protective coating can be applied in just days, reducing facility downtime and installation costs while allowing for regular inspection and replacement.
This X-ray backscatter imaging device acquires depth-sensitive information from an object during a single scan of one side to generate high-resolution 2D and 3D images of the internal structure of the object. Compton scatter imaging is an imaging technique that uses photon scattering to scan an object for depth-sensitive information and create images of it based on this information. In X-ray backscatter imaging, a subset of Compton scatter imaging, the photon source and detector are on the same side, allowing the device to obtain internal images of an object even when only one side is accessible. This feature of X-ray backscatter imaging both reduces the invasiveness of imaging for medical or security purposes and broadens its application to imaging scenarios where access to more than one side of the object is not possible. However, the limitations on signal acquisition from a single side prevent the application of conventional X-ray backscatter imaging to 2D and 3D tomography, which means that available XBI systems can obtain only 2D images in a single scan.
Researchers at the University of Florida have developed an X-ray backscatter imaging device that overcomes these limitations by using spectral detection, which allows the device to collect depth-sensitive information in a single, one-sided scan sufficient to generate both 2D and 3D images. This X-ray backscatter imaging device requires just one scan for each image type, decreasing scanning time and reducing the imaged object’s exposure to radiation.
X-ray backscatter imaging device that rapidly produces high-resolution 2D and 3D images for use in emergency medical situations, veterinary medicine or many industrial testing scenarios
X-ray backscatter imaging (XBI), in contrast to traditional X-ray imaging, generates an image using radiation reflected back from the object, called the backscatter pattern, rather than X-rays that pass through it. Since the backscatter pattern is dependent on the properties of the object, it contains the information required to generate an image. However, X-ray backscatter imaging typically generates only a 2D image because of limitations on signal acquisition that restrict the amount of depth-sensitive information acquirable in a single scan. This X-ray backscatter imaging device overcomes the limits on signal acquisition by using spectral detection, which involves binning the collected backscatter signal into separate energy groups to obtain depth sensitive information. This information, combined with an image reconstruction algorithm, allows the device to generate high-resolution 2D and 3D images, where at least one dimension corresponds to depth in the object.